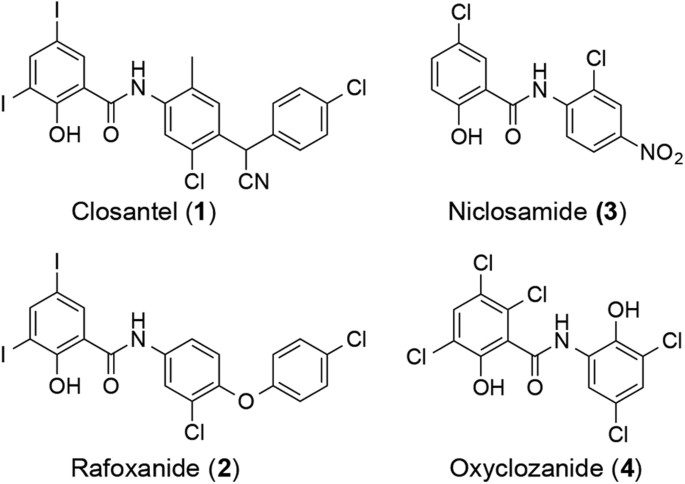
05 Jan Anthelmintics Explained
Anthelmintics are drugs that cure parasitic worm infections. This includes both flat worms like flukes and tapeworms and circular worms like nematodes. They play an essential role in both human tropical medicine and veterinary medicine. According to the World Health Organization, 2 billion people are infested with parasitic worms. Parasitic worms also infect animals and crops, resulting in decreased food production and economic losses. The infection of household pets is also significant. Indeed, the companion animal market is a key commercial concern for animal health enterprises performing pharmaceutical development projects.
Despite the prevalence of parasitic worms, anthelmintic medicine research is a poor cousin of the pharmaceutical sector. The primary reason for this is that the nations most impacted by these tropical diseases have little money to invest in drug research or therapy. It should come as no surprise, then, that the medications presently available for human use were first developed for animal use. As a result, chemotherapeutic medication treatment choices are pitifully restricted. This predicament has been exacerbated in some respects by ivermectin’s phenomenal success over the last twenty years, which has lowered motivation for anthelmintic drug research projects. The same is cause for concern because anthelmintic resistance has been reported regularly in animals, and it may only be a matter of time until similar phenomenon manifests in human parasites.
Classes of antihelmintics
Anthelmintics are categorised based on their chemical makeup and mode of action. There are just a few primary classes, which are discussed briefly below. The majority of data on the physiological and pharmacological actions of anthelmintics has come from studies on the large parasitic worm A. suum, however C. elegans has been beneficial in defining molecular targets.
Piperazine
Piperazine was first used as an anthelmintic in the 1950s, and it is now an active element in children’s over-the-counter thread worm remedies. Its mode of action has been extensively studied in A. suum. Surprisingly, little study has been conducted on its function in C. elegans, and there is no indication that it functions differently than in A. suum. In A. suum, it acts as a moderate GABA-mimetic, inducing flaccid, reversible paralysis of body wall muscle. According to single channel studies, it is a low-effective, partial agonist at GABA-gated chloride channels.
Thiabendazole
Thiabendazole was the first member of this class to be found in 1961, and a number of additional benzamidazoles were later approved as broad-spectrum anthelmintics. There is a substantial corpus of research on these compounds that indicates a wide range of biological effects. Nonetheless, it is clear that their anthelmintic activity is linked to their ability to disrupt the cytoskeleton through a particular interaction with -tubulin. The effects of benzimidazoles on C. elegans, including as reduced movement and reproduction, as well as a detrimental effect on oocytes, are consistent with disruption of activities that require integral microtubules. The sensitivity of C. elegans to benzimidazoles is controlled by a single gene, ben-1, which encodes -tubulin (Driscoll et al., 1989). This has laid the groundwork for future studies into the molecular basis of benzimidazole resistance in parasitic worms. It has been demonstrated that benzimidazole resistance in Haemonchus contortus is associated with the presence of certain -tubulin alleles in drug resistant isolates (Kwa et al., 1994). Experiments were carried out to see if a specific -tubulin isoform could confer resistance to the drug, and the results showed that expressing an H. contortus allele of -tubulin from benzimidazole susceptible isolates, but not the allele present in resistant isolates, can rescue the sensitivity of C. elegans ben-1 mutants to the drug. This clearly demonstrated that anthelmintic resistance may be conferred by a single amino acid alteration in -tubulin, Y for F. This is the first elegant example of a’model hopping’ strategy in which the genetic tractability of C. elegans is utilized directly to characterize gene function in a parasitic worm.
Morantel, pyrantel, and levamisole
These anthelmintics function as nicotinic receptor agonists, causing spastic muscular paralysis by activating excitatory nicotinic acetylcholine (nACh) receptors on body wall muscle (Aceves et al., 1970; Aubry et al., 1970). Their unique mode of action on A. suum body wall muscle preparation has been extensively investigated at the single-channel level (see Martin et al., 2005 for review). Pharmacological research has shown three nACh receptor subtypes: an N-type (preferentially activated by nicotine), a B-type (preferentially activated by bephenium), and an L-type (preferentially activated by bephenium) (preferentially activated by levamisole and associated with levamisole resistance). Levamisole and related compounds cause spastic paralysis and egg-laying in C. elegans. Indeed, recordings from C. elegans body wall muscle using levamisole and nicotine as agonists have provided more evidence that nACh receptor muscle subtypes with various nACh receptor subunit compositions exist. At least four subunits make up the levamisole receptor: unc-38, unc-29, unc-63, and lev-1. As a result, these anthelmintics may be used to deconstruct the subtypes and stoichiometries of native nematode nicotinic receptors.
Perhaps most importantly, levamisole has demonstrated good performance in forward genetic screening. Tetramisole was first used in the studies, but it was subsequently replaced by the more active isomer, levamisole. Throughout the last two decades, these screens have yielded a pool of mutants that have been used to assign function to genes expressed at the neuromuscular junction. Some of them are nicotinic receptor subunits, while others are not and contribute to regulate either nicotinic receptors or
Paraherquamide
Both paraherquamide A and marcfortine A are oxindole alkaloid members found in Penicillium paraherquei and Penicillium roqueforti. Marcfortine A was revealed to be active against C. elegans in a high throughput screen. In a C. elegans membrane preparation, a specific, high affinity binding site for paraherquamide was discovered, with an apparent Kd of 263nM. In parasitic worms, paraherquamide and its derivative, 2-deoxy-paraherquamide, produce flaccid paralysis in vitro. In vitro pharmacological testing of these drugs on acetylcholine-stimulated body wall muscular contractions in A. suum muscle strips revealed that they behave as typical competitive antagonists, shifting concentration-response curves to the right in a parallel fashion. These drugs have no effect on A. suum body wall muscle tension or membrane potential. Paraherquamide also inhibits other nicotinic agonists, but not equally. This antagonist appears to distinguish nicotinic receptor subtypes on the muscle and has a greater affinity for receptors mediating the response to levamisole and pyrantel than for receptors mediating the response to nicotine. As a result, paraherquamide is expected to be a strong antagonist of the levamisole-selective receptor on C. elegans body wall muscle. Importantly, unlike other well-known drugs that interfere with cholinergic transmission, such as levamisole, this anthelmintic family works as competitive antagonists rather than cholinomimetics. Despite the fact that the use of paraherquamide in forward genetic screening has yet to be documented, it has the potential to provide interesting new mutants. Mutations that increase transmitter release should provide resistance since it is a competitive inhibitor of the nACh receptor in the body wall. As a result, other negative regulators of neurotransmitter release may be discovered by a forward genetic search.
Ivermectin
In the 1980s, Merck introduced ivermectin as an anthelmintic. It is a semi-synthetic avermectin derivative, a large macrocyclic lactone fermentation product of Streptomyces avermitilis. Its activity is exceptionally powerful (1nM) and long-lasting, prompting other companies to invest in the development of ivermectin analogues such as moxidectin, milbemycin oxime, doramectin, selamectin, abamectin, and eprinomectin. In this investigation, C. elegans was employed to look for new macrocyclic lactones having ivermectin-like activity.
Ivermectin paralyzes the nematode’s pharyngeal and body wall muscles for a long time. It has been shown to interact with 7 nACh receptors, acetylcholine-gated chloride channels, GABA-gated chloride channels, histamine-gated chloride channels, glycine receptors, and P2X4 receptors (Krause et al., 1998). Its potent antihelmintic activity, however, is linked to its affinity for nematode glutamate-gated chloride channels (GluCl). The Merck team defined this after successfully cloning the GluCl and GluCl ion channel subunits from C. elegans. Both components were expressed independently or concurrently in Xenopus oocytes. GluCl interacts with micromolar ivermectin but not with glutamate, whereas glutamate does not react with ivermectin. GluCl and GluCl co-expression resulted in a glutamate-responsive channel that is allosterically controlled by nanomolar ivermectin. Following that, researchers uncovered a small family of worm genes that produce GluCl channels. The name is puzzling because the same genes were discovered using both homology and forward genetic screens for ivermectin resistance genes. GluCl subunits are produced by four C. elegans genes, two of which are alternatively spliced to form GluCl channels: GluCl1 encoded by glc-1; GluCl2A and B encoded by avr-15; GluCl3A and B encoded by avr-14; and GluCl4 expressed by glc-3. Glc-2 encodes just one GluCl component, and another gene, glc-4, is separate from the genes encoding and subunits. Although the pharmacology of channels built from these GluCl subunits has been reported in heterologous expression techniques, the important subject of subunit stoichiometry and native channel pharmacology remains unsolved. Further study on C. elegans is deciphering the expression pattern of GluCl subunits throughout the nervous system, providing a more complete picture. Avr-15 and glc-2 are expressed in the pharyngeal muscle, for example. As a consequence, GluCl2 and GluCl subunits may co-assemble to form a native ivermectin sensitive channel. It is unknown whether or not other subunits contribute to the functioning receptor. The pharyngeal muscle of avr-15 mutants, on the other hand, does not respond to ivermectin, indicating that GluCl2 is involved. Ivermectin does not block avr-15 mutant pharynxs, but populations of avr-15 mutants exposed to it are paralyzed. As a result, the paralytic activity is not mediated via GluCl channels in the pharynx. This may also be true for parasitic nematodes. Ivermectin, for example, has an anthelmintic impact on Ascaridia galli but does not clog its pharynx. It may be more advantageous to investigate the role of GluCl channels in the nervous system in order to gain a better understanding of their role in mediating the paralytic effects of ivermectin. The majority of information on avr-14 and avr-15 is currently available. These genes are expressed in the motor nervous system, and GluCl3A and B immunostaining in H. contortus motor neurons. In C. elegans, these GluCl channels affect the duration of forward movement, which is a well-known glutamatergic-regulated activity. The presence of H. contortus GluCl3 subunits in C. elegans avr-14 mutants restores the wild type movement pattern, indicating that this function is conserved between C. elegans and H. contortus. Ivermectin’s paralytic action is most likely owing to its strong activation of GluCl in worm motornervous systems. However, the precise role of individual GluCl channels in mediating the actions of ivermectin on these circuits is uncertain. C. elegans’ ivermectin resistance mechanism has also been investigated. High level resistance is a complex phenomenon requiring mutations in at least three genes: glc-1, avr-14, and avr-15. Other genes that have been implicated include those that control membrane permeability (osm-1) and gap junctions (unc-7 and unc-9). In the field, the role of GluCl mutations in conferring ivermectin resistance to parasitic nematodes is a more complex and disputed issue.
Emodepside
The semi-synthetic cyclodepsipeptide chemical emodepside is generated from PF1022A, a fermentation product obtained from the Camelia japonica fungus Mycelia sterilia. Its discovery and antihelmintic capabilities have lately been investigated (Harder, von Samson-Himmelstjerna, 2002). It works against parasite isolates that are resistant to benzimidazole, levamisole, and ivermectin, showing that it has a distinct mode of action. Although the molecule has pore-forming characteristics in planar lipids, this does not appear to be important in conferring anthelmintic potency because an optical isomer of emodepside with comparable pore-forming capabilities has no anthelmintic impact. As a result, it appears to work by attaching to a receptor in a stereospecific manner. A. suum has shown muscle paralysis, indicating a calcium- and potassium-dependent mechanism of action. A putative receptor for the cyclodepsipeptides was cloned from an H. contortus cDNA library after immunoscreening with an antibody against PF1022 A. This receptor, known as HC110R, has been reported to gate calcium flow in HEK293 cells in a PF1022 A-dependent manner. Mammalian latrophilins are G protein-coupled receptors that bind the neurotoxic latrotoxin. Because latrotoxin paralyzes humans by inducing neurotransmitter release, the identification of latrophilin as an emodepside receptor raised the intriguing possibility that emodepside may cause nematode paralysis by inducing excessive neurotransmitter release at neuromuscular sites.
C. elegans is particularly susceptible to the effects of emodepside at nanomolar concentrations (Bull et al., 2007). Slower development, reduction of pharyngeal pumping, decreased motility, and inhibition of egg-laying all contribute to ‘bagging’ in mature hermaphrodites. Thus, C. elegans has provided an appropriate model for determining the molecular target, or targets, via which emodepside exerts its pleiotropic effects, as well as investigating the involvement of latrophilins.
Potential latrophilins are encoded by two genes in C. elegans, lat-1 and lat-2. RNAi and gene knockouts were utilized to investigate the role of these latrophilins in mediating emodepside’s inhibitory effects on feeding and motility. The pharyngeal system of Lat-1 mutants is less responsive to emodepside, but their locomotor activity is reduced equivalent to that of wild-type animals. Because lat-2 mutants act similarly to wild-type animals, emodepside has no effect on locomotion via the second latrophilin, LAT-2. In terms of emodepside’s impact on locomotion, there is likewise no functional redundancy between LAT-1 and LAT-2, because the double mutant lat-2,lat-1 reacts to emodepside in the same way as the single mutant, lat-1. Emodepside has obviously latrophilin-independent actions.
Forward genomic scans have recently revealed new details about how emodepside inhibits C. elegans muscle. A search across 20,000 genomes for mutants that could move and reproduce on micromolar emodepside produced nine alleles of a single gene, slo-1. Strains having functional null alleles of slo-1 are especially resistant to the inhibitory effects of emodepside on locomotion and feeding. Furthermore, slo-1 gain-of-function alleles behave similarly to emodepside-treated animals but are not hypersensitive to emodepside. These data provide credence to the concept that emodepside impairs neuromuscular activity through a SLO-1-dependent mechanism.
SLO-1 is a calcium-activated potassium channel that works in the same way that mammalian BK channels do. As a result, the discovery of SLO-1 as a primary emodepside effector is consistent with recent study on Ascaris muscle, which indicated calcium and potassium-dependent hyperpolarisation. This channel is highly conserved throughout animal phyla and is required for the regulation of neuronal and muscle cell excitability. In C. elegans, it is widely produced in the nervous system and body wall muscle, but not in the pharyngeal muscle. It has been established that emodepside can inhibit eating when SLO-1 is present in neurones or body wall muscle by creating a wild-type copy of slo-1 in specific subsets of cells in a slo-1 null background. Previous study discovered that mutations in a number of genes that produce synaptic proteins impart varying sensitivity to emodepside’s effect on eating, lending credence to the idea that it works in the brain network to disrupt rhythmic feeding behavior.
http://www.mhsvbrstudy.com/2020/01/14/fenbendazole-dosage-guide/
Nitazoxanide
Nitazoxanide, an inhibitor of pyruvate ferredoxin oxidoreductase, is effective against a wide variety of intestinal protozoa and helminths. It is now employed in the treatment of protozoal diseases. This chemical’s mode of action in nematodes has not been established, however anaerobic electron transport enzymes may be a plausible target. The effect of nitazoxanide on the growth and development of C. elegans has been investigated. Nitazoxanide 100 M decreased population growth by just 33% after seven days of culture. Mebendazole (5 M) and albendazole (1 M), on the other hand, reduced growth by more than 90%. Nitazoxanide had no effect on Heligmosomoides polygyrus embryonation or hatching at 100 M. As a result, as compared to other anthelmintic medications, the efficacy of this molecule is rather low.
http://www.mhsvbrstudy.com/2020/01/13/fenbendazole-for-humans/
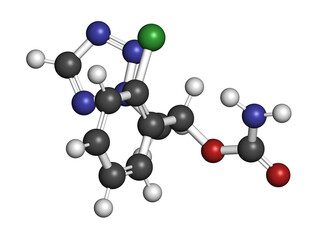
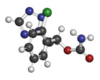
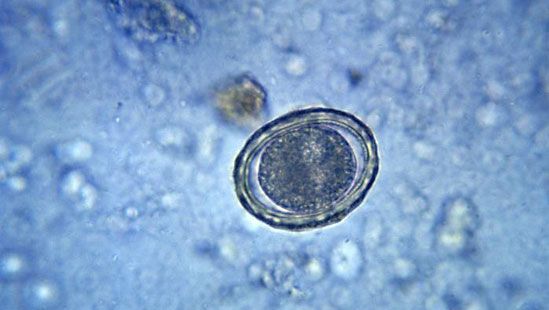
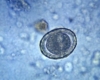
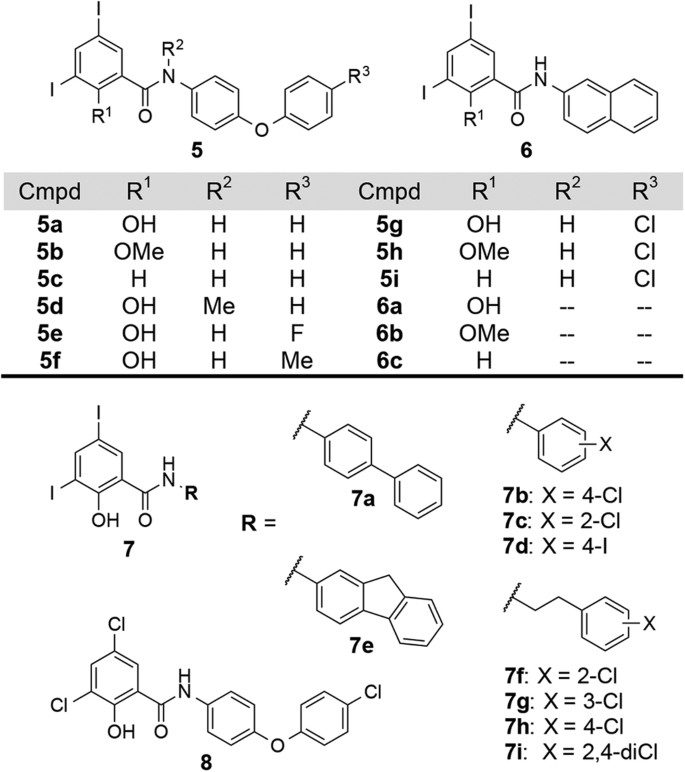
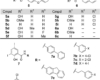
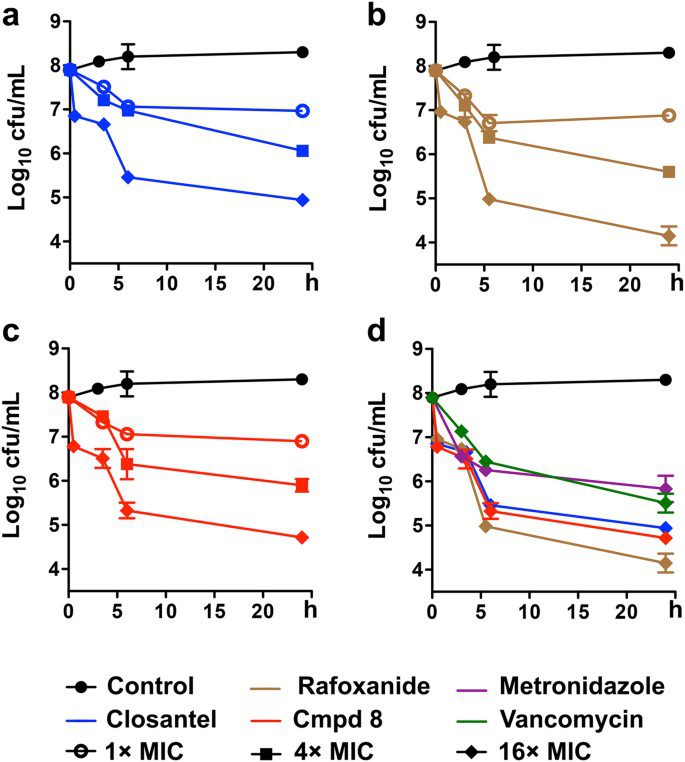
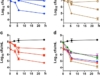
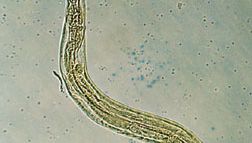
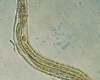
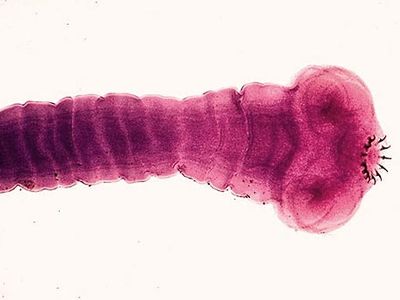
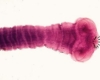
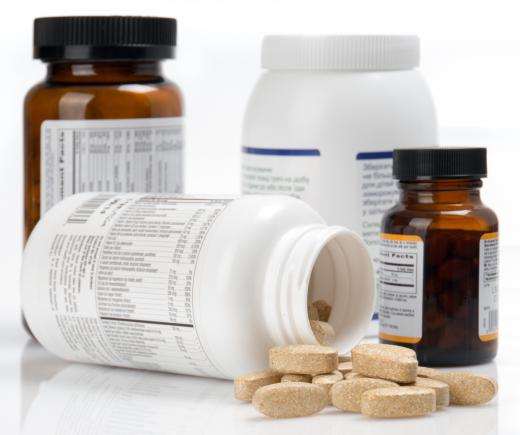
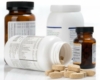
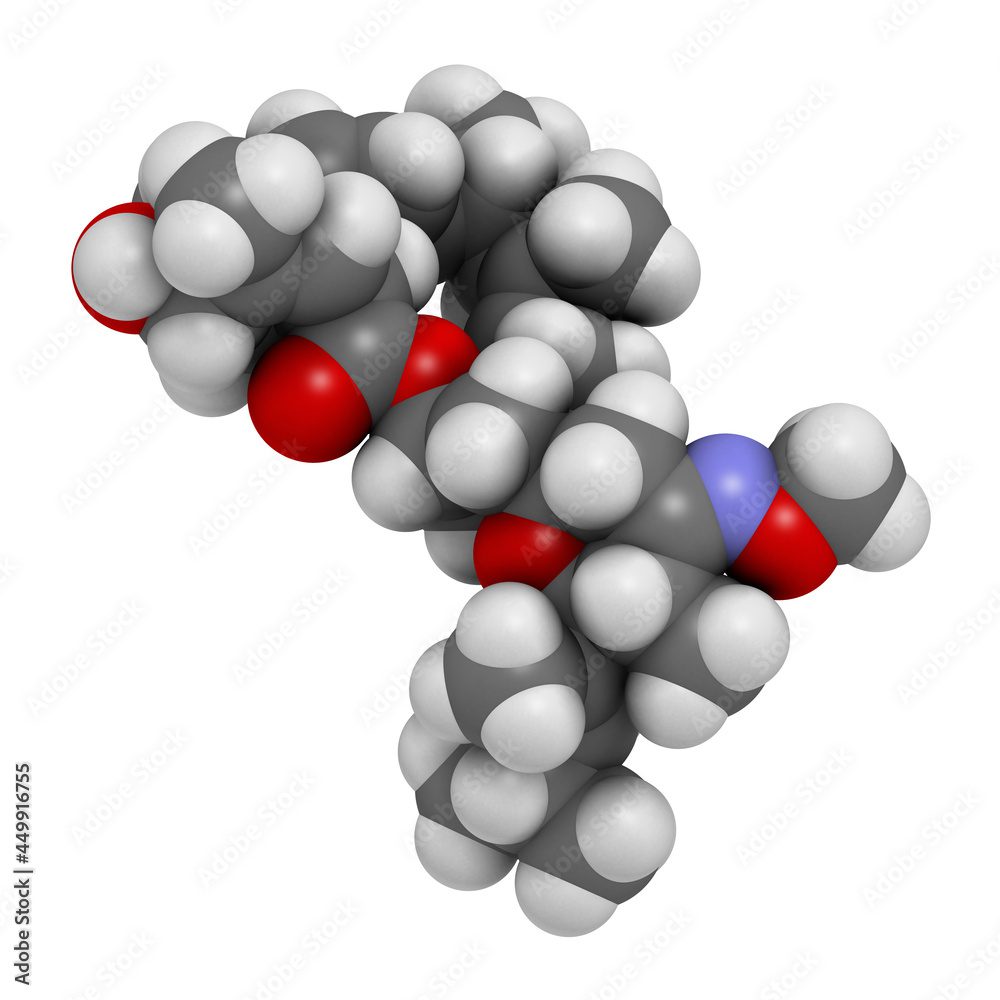
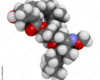